The Electromagnetic Spectrum
More about the Electromagnetic Spectrum
As it was explained in the Electromagnetic Spectrum - Level 1 of
Imagine the Universe!,
electromagnetic
radiation can be described in terms of a stream of photons, each
traveling
in a wave-like pattern, moving at the
speed of
light and carrying some amount of energy. It was pointed out that
the only
difference between radio
waves, visible
light, and
gamma-rays is
the energy of the photons. Radio waves have photons with low energies,
microwaves
have
a little more energy than radio waves,
infrared has
still more, then visible,
ultraviolet,
X-rays, and
gamma-rays.
Actually, the amount of energy a photon has makes it sometimes behave more
like a wave and sometimes more like a particle. This is called the
"
wave-particle duality" of light. It is important to understand that we are not talking about a difference in what
light IS, but only in how it behaves. Low energy photons (such as radio) behave more like
waves, while higher energy photons (such as X-rays) behave more like particles.
This is an important difference for scientists to know when they design
detectors and telescopes to try to 'see' EM radiation from very low to very
high energies. In fact, scientists choose whichever description of light they
need for their study.
The truth is, the
electromagnetic
spectrum can be expressed in terms of energy,
wavelength, or
frequency. Each
way of thinking about the EM spectrum is related to the others in a precise
mathematical way. The relationships are:
the wavelength equals the speed of light divided by the frequency
or
lambda = c / nu
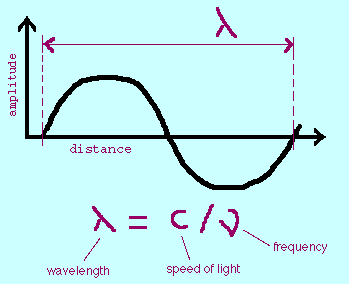
and
energy equals
Planck's
constant times the frequency
or
E = h x nu
(lambda and nu are just letters from the Greek alphabet that scientists like
to use rather than l or f. It just helps them to keep things straight!) Both the
speed of light and Planck's constant are, well, constant: They never change
their values. Ever. The speed of light is equal to 299,792,458 m/s (186,212
miles/second). Planck's constant is equal to 6.626 x 10-27
erg-seconds.
Show me a chart of the wavelength, frequency, and energy regimes
of the spectrum !
Space Observatories in Different Regions of the EM Spectrum
Radio observatories

Radio waves CAN make it through the Earth's atmosphere without significant
obstacles (In fact radio telescopes can observe even on cloudy days!).
However, the availability of a space radio observatory complements
radio telescopes on the Earth in some important ways.
There are a number of radio observatories in space. These include Polar, Cluster II,
ISEE 1, ISEE 2, GOES 9 and Voyager 1. Most of these study the
ionospheres of the planets down to 3 x 10-4 Hz. Some have
been used to monitor radio signals given off by earthquakes.
One is a special technique used in radio
astronomy called
"interferometry". Radio astronomers can combine data from two
telescopes that are very far apart and create
images which have
the same
resolution as if they had a single telescope as big as the distance
between the two telescopes! That means radio telescope arrays can see
incredibly small details. One such array is called the Very Large Baseline
Array (VLBA): it consists of ten radio telescopes which reach all the way from
Hawaii to Puerto Rico: nearly a third of the way around the world! By
putting a radio telescope in
orbit around the
Earth, radio astronomers could make images as if they had a radio telescope
the size of the entire planet! The Very Long Baseline Interferometry
(VLBI) Space Observatory Program (VSOP) attempts to do just that. This
Japanese mission was launched in February 1997, and renamed
HALCA shortly thereafter.

Microwave observatories
The sky is a source of microwaves in every direction, most often called the
microwave background. This background is believed to be the remnant from the
"Big Bang" scientists believe our Universe began with. It is
believed that a very long time ago all of space was scrunched together in a
very small, hot ball. The ball exploded outward and became our Universe as it
expanded and cooled. Over the course of the past several billion years (the
Universe's actual age is still a matter of debate, but is believed to be
somewhere between ten and twenty billion years), it has cooled all the way to
just three degrees above zero. It is this "three degrees" that we
measure as the microwave background.
From 1989 to 1993 the
Cosmic Background Explorer (COBE) (http://lamda.gsfc.nasa.gov/), made
very precise measurements of the temperature of this microwave
background. COBE mapped out the entire microwave background,
carefully measuring very
small differences in temperatures from one direction to another. Astronomers
have many theories about the beginning of the Universe and their theories
predict how the microwave background would look. The very precise measurements
made by COBE eliminated a great many of the theories about the Big Bang.
The Wilkinson Microwave Anisotropy Probe (WMAP), launched in the summer of 2001, measures the temperature fluctuations of the cosmic microwave background
radiation over the entire sky with even greater precision. WMAP is
answering such fundamental questions as:
Tell me more about WMAP !

Infrared observatories
The most recent
infrared
observatory currently in orbit was the
InfraredSpace Observatory (ISO) (http://isowww.estec.esa.nl/), launched in November 1995 by the European Space
Agency, and operated until May 1998. It was placed in an elliptical orbit with a 24 hour period
which kept it in view of the ground stations at all times, a necessary
arrangement since ISO transmitted observations as it made them rather than
storing information for later playback. ISO observed from 2.5 to
240 microns.
In August 2003, NASA launched the Space
Infrared Telescope Facility (SIRTF). SIRTF uses an passive cooling
system (i.e. it radiates away its own heat rather than requiring an
active refrigerator system like most other space infrared observatories) and
it was placed in an earth-trailing, heliocentric orbit, where it will not have to contend with Earth
occultation of
sources nor with the comparatively warm environment in near-Earth space.
Another major infrared facility coming soon will be the Stratospheric
Observatory for Infrared Astronomy (SOFIA). Although SOFIA will not be an
orbiting facility, it will carry a large telescope within a 747 aircraft flying
at an altitude sufficient to get it well above most of the Earth's infrared
absorbing atmosphere. SOFIA will be replacing the Kuiper Airborne
Observatory.

Visible spectrum observatories
The only visual observatory in orbit at the moment is the Hubble Space
Telescope (HST). Like radio observatories in space, there are
visible
observatories already on the ground. However, Hubble has several special
advantages over them.
HST's biggest advantage is, because it is above the Earth's atmosphere, it
does not suffer distorted vision from the air. If the air was all the same
temperature above a telescope and there was no wind (or the wind was perfectly
constant), telescopes would have a perfect view through the air. Alas, this is
not how our atmosphere works. There are small temperature differences, wind
speed changes, pressure differences, and so on. This causes
light passing
through air to suffer tiny wobbles. It gets bent a little, much like light
gets bent by a pair of glasses. But unlike glasses, two light beams coming
from the same direction do not get bent in quite the same way. You've probably
seen this before -- looking along the top of the road on a hot day, everything
seems to shimmer over the black road surface. This blurs the image
telescopes see, limiting their ability to resolve objects. On a good night in
an observatory on a high mountain, the amount of distortion caused by the
atmosphere can be very small. But the Space Telescope has NO distortion from
the atmosphere and its perfect view gives it many many times better resolution
than even the best ground-based telescopes on the best nights.
Another advantage of the Space Telescope is that without the atmosphere in
the way, it can see more than just the visible
spectrum. The
Space Telescope can also see
ultraviolet
light which normally is absorbed by the Earth's atmosphere and cannot be seen
by regular telescopes. So the Space Telescope can see a much wider portion of
the spectrum.

Ultraviolet observatories
Right now there are no dedicated ultraviolet observatories in orbit. The
Hubble Space Telescope can perform a great deal of observing at ultraviolet
wavelengths,
but it has a very fairly small field of view. Until September
1996, the International Ultraviolet Explorer (IUE) was operating and observing
ultraviolet radiation. Its demise, although unfortunate, was hardly premature:
IUE was launched in January, 1978 with planned operations of three years. IUE
functioned more or less like a regular ground based observatory save that
the telescope operator and scientist did not actually visit the telescope, but
sent it commands from the ground. Other than some care in the selection of
materials for filters, a UV telescope like IUE is very much like a regular
visible light telescope.
In addition to IUE, there have been fairly important recent UV space
missions. A reusable shuttle package called Astro has been flown twice in the
cargo bay of the space shuttle: it consisted of a set of three UV telescopes.
Unlike HST, the Astro UV telescopes had very large fields of view and so could
take images of larger objects in the sky -- like galaxies. For instance, if
the Hubble Space Telescope and the Astro telescopes were used to look at the
Comet Hale-Bopp, Hubble would be able to take spectacular pictures of the core
of the comet. The Astro telescopes would be able to take pictures of the
entire comet, core and tail.

Extreme Ultraviolet observatories
There are two extreme ultraviolet observatories in space at the moment.
One of them is the very first extreme ultraviolet observatory ever, the
ExtremeUltraviolet Explorer (EUVE) (http://www.cea.berkeley.edu/).
Astronomers have been somewhat reluctant to explore from space at the extreme
ultraviolet wavelengths since all theory strongly suggests that the
interstellar
medium (the tiny traces of gases and dust between the
stars) would absorb
radiation in this portion of the spectrum. However, when the Extreme
Ultraviolet Explorer (EUVE) was launched, observations showed that the solar
system is located within a bubble in the local interstellar medium. The region
around the Sun is relativity devoid of gas and dust which allows the EUVE
instruments to see much further than theory predicted.
Another extreme ultraviolet observatory currently operating is the
Array of Low Energy X-ray Imaging Sensors (ALEXIS) (http://nis-www.lanl.gov/nis-projects/alexis/). Although its name
indicates that it is an
X-ray observatory,
the range of energy ALEXIS is exploring is at the very lowest end of the
X-ray spectrum and often considered to be extreme ultraviolet.
ALEXIS was launched on 25 Apr 1993 on a Pegasus rocket. During
launch, a hinge plate supporting one of the solar panels broke.
However, the satellite survived, and the panel remains
connected to the satellite via the electrical cables and a
tether, and it still provides the requisite power to the satellite.
ALEXIS is spinning about an axis pointed approximately toward the
sun. ALEXIS provides
sky maps on a daily basis whenever the satellite is not in a 100%
sunlight orbit. These sky maps are used to study diffuse x-ray
emission, monitor the brightness of known EUV objects, and to detect
transient objects.

X-ray observatories
There are several X-ray observatories currently operating in space with
more to be launched in the next few years.
The
Rossi X-ray Timing Explorer
(RXTE) was launched on December 30, 1995. RXTE is able to make very
precise timing measurements of X-ray objects, particularly those which show
patterns in their X-ray emissions over very short time periods, such as certain
neutron star
systems and
pulsars.
Other X-ray observatories currently operating in space include
ROSAT, a joint venture between
the United States, Germany, and the United Kingdom; the
Advanced Satellite for Cosmology
and Astrophysics (ASCA), a joint U.S.-Japan venture; the
Kvant astrophysics module
attached to the Russian space station Mir, and
Beppo SAX, an Italian X-ray satellite.
NASA launched another major new X-ray astronomy satellite,
the
Chandra X-ray Observatory (CXO), in mid 1999.

Gamma-ray observatories
The
Compton Gamma-Ray Observatory (CGRO) was launched by the space shuttle in
April 1991. The observatory's instruments are dedicated to observing the
gamma-ray sky, including locating
gamma-ray burst
sources, monitoring
solar flares,
and other highly energetic astrophysical phenomenon. An unexpected discovery
which Compton has made was the observation of gamma-ray burst events coming
from the Earth itself at the top of thunderstorm systems. The cause of this
phenomenon is not known, but it is currently suspected to be related to
"Sprites": lightning flashes which are occasionally seen jumping
upward from cloud tops to the upper stratosphere.
The Russian gamma-ray observatory
Granat has exhausted its
control fuel. Its last maneuver in 1994 was to initiate a roll which
allowed it to perform a continuous all-sky survey until Nov 1998.
The European mission INTEGRAL was launched in October 2002. It
is studying gamma-ray bursts, and sources within our galaxy.
The next major gamma-ray missions in the near future include SWIFT
and the Gamma-Ray Large Area Space Telescope (GLAST). SWIFT will
study gamma-ray bursts, and be capable of quickly pointing narrow
field X-ray and optical detectors in the direction of gamma-ray bursts
detected by its large field detectors. GLAST will have
a field of view twice as large as that of the Compton Gamma-Ray
Observatory, and a sensitivity of up to 50 times greater than
Compton's EGRET instrument. GLAST will study a wide range of
gamma-ray objects, including pulsars, black holes, active
galaxies, diffuse gamma-ray emission, and gamma ray bursts.
|